Biomedical abnormalities in Autism Spectrum Disorder
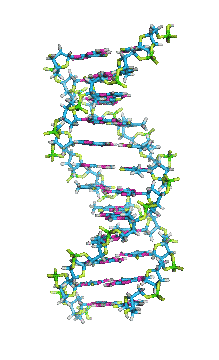
Scientific studies listed below indicate that children with ASD suffer from a range of dysfunctions including: genetic, neurological, gastrointestinal, neurotransmitter, hormonal, immune function, detoxing and oxidative stress systems. We discuss what can be done to redress some of these to restore function and improve symptoms.
There is a broad consensus amongst scientists that Autism Spectrum Disorder is caused by genetic vulnerabilities interacting with environmental factors. The genetic vulnerabilities can be either inherited, meaning that they run within the family, or de-novo (new) mutations that were not inherited but most likely caused by environmental toxins in utero. The environmental factors are lack of nutrients in our "Western style diet" of highly processed foods, high in sugar and refined carbohydrates and depleted of key minerals and vitamins. In addition environmental toxins, pesticides, chemicals and even food colourings, additives and preservatives can cause malfunction in cells. The European union passed a law in 2008 that any foods containing some of these chemicals must have a red label warning which reads "Contains substances that cause hyperactivity and behaviour problems." Consequently these food colourings, additives and preservatives have disappeared from European supermarkets.
The following list is a brief summary of some of the findings from research into abnormal biological markers in people with autism. Click on a heading to go to the research area. Click on any numbered reference to be directed to the research paper. Note Dr. Duff’s remarks in Italics.
-
Neurological abnormalities; Epilepsy and Seizures;
-
Gastrointestinal problems;
-
Neurotransmitter abnormalities;
-
Hormonal abnormalities;
-
Auditory, visual, tactile and oral sensory processing disorders and motor difficulties;
-
Reduced cerebral blood flow and cerebral oedema;
-
Abnormality of the immune function and chronic inflammation;
-
Oxidative stress;
-
Mitochondrial dysfunction.
-
What does it all mean and what can be done?
1. Neurological abnormalities in Autism Spectrum Disorders:
Various neuropathological and MRI studies have pointed to a number of neurological abnormalities in autism:
-
Significantly elevated calcium levels in autistic brains compared to controls, followed by elevations of mitochondrial aspartate/glutamate carrier rates and mitochondrial metabolism rates [1].
-
Abnormal neuronal migration in both brainstem and cerebellum and disorganised columns of neurons in the cerebral cortex. (Omega 3, DHA controls migration of stem cells to the cortex during neurogenesis)
-
Significant reduction in granular and Purkinje cell numbers, often accompanied by gliosis (proliferation of astrocytes in damaged areas of the brain).
-
Marked active/ongoing neuroglial activation and neuroinflammation (Omega 3, EPA reduces inflammation)
-
Abnormalities of cortical development has been observed in some cases, including areas of Increased cortical thickness, high neuronal density, neuronal disorganization and poor differentiation of neurons. (Omega 3, DHA controls migration of stem cells to cortex during neurogenesis)
-
Abnormalities in brain size and volume, recently linked to increased tissue water content in brain matter
-
Reduced blood flow to parts of the brain (Due to reduced brain activity)
-
Abnormalities in the size and density of neurons and less dendritic branching, with increased neuron density and shorter connecting fibres, pointing to delays in neuronal maturation(associated with low Omega 3, DHA status?).
-
Autoantibodies to brain proteins, notably to myelin basic protein, neuron-axon filament protein and glial fibrillary acidic protein.
[1, 2, 3, 4, 5, 6, 7,8, 9, 10, 11, 12, 13, 14]
Epilepsy and Seizures in Autism
Approximately 1 in 3 children with autism develop seizures in childhood or during adolescence, a serious problem considering that epilepsy, in addition to immunological problems, more than doubles the risk of early death in individuals with autism compared to healthy population. One longitudinal study found that of the 120 individuals with autism followed, the death rate was 5.6 times higher than expected at the time of follow-up. Epilepsy has been found to be a major negative factor on cognitive, adaptive and behavioural/emotional outcomes for individuals with autism.
2. Gastrointestinal abnormalities in autism Spectrum Disorders
Individuals with autistic spectrum disorders tend to suffer from various, sometimes severe gastrointestinal problems. Children with ASD are suspected to have a higher rate of gastrointestinal (GI) symptoms when compared with children of either typical development or another developmental disorder, although large-scale studies are yet to be carried out and the significance of the association between many gastrointestinal pathologies and autism is yet to be confirmed.
A recent expert-consensus report on evaluation, diagnosis, and treatment of gastrointestinal disorders in individuals with autism concluded that care providers should be aware that problem behaviour in patients with ASDs may be the primary or sole symptom of the underlying medical condition, including gastrointestinal disorders, and guidelines have been issued on evaluation and treatment of common gastrointestinal problems in children with ASDs, such as abdominal pain, chronic constipation, and gastroesophageal reflux disease [20, 21].
The most frequent complaints are chronic constipation and/or diarrhea (frequently accompanied byindigested or partially digested food in stools), gaseousness, and abdominal discomfort and distension (irritable bowel syndrome)[22].Decreased sulfation capacity of the liver, (affecting detoxification)pathologic intestinal permeability(allowing toxins and antigens to enter the blood stream), increased secretory response to intravenous secretin injection, and decreased digestive enzyme activities were reported in many children with autism. Treatment of digestive problems is reported to have positive effects on autistic behaviour in some individuals [23, 24, 25].
Defects of innate immune responses in ASD children with GI problems have been detected, and intestinal pathology, including ileocolonic lymphoid nodular hyperplasia (LNH) and mucosal inflammation, with enhanced pro-inflammatory cytokine production, has been characterised in various studies. A majority of the children were shown to have chronic swelling of the lymphoid tissue lining the intestines, particularly near where the small and large intestines meet, and chronic inflammation of the large intestine. Secondary eosinophilic colitis has also been observed in autism. There is a consistent profile of CD3+ lymphocyte cytokines in the small and large intestinal mucosa of these ASD children, involving increased pro-inflammatory and decreased regulatory activities [26, 27, 28, 29, 30].The mucosal immunopathology in children with autism is reported to be suggestive of autoimmune lesion and is apparently distinct from other inflammatory bowel diseases [31, 32].
One study examining histological findings in children with autism revealed high incidence of grade I or II reflux esophagitis, chronic gastritis and chronic duodenitis. The number of Paneth cells in the duodenal crypts was significantly elevated in autistic children compared to controls. Low intestinal carbohydrate digestive enzyme activity was reported in over half of the children with autism, although there was no abnormality found in pancreatic function. Seventy-five percent of the autistic children had an increased pancreatic-biliary fluid output after intravenous secretin administration, suggesting an upregulation of secretin receptors in the pancreas and liver [33].
There are also reports of prominent epithelium damage (as would be caused by wheat lectins (WGA)[34], and of significant alterations in the upper and lower intestinal flora of children with autism. One striking finding was complete absence of non-spore-forming anaerobes and microaerophilic bacteria from control children and significant numbers of such bacteria from children with autism. The faecal flora of ASD patients was found to contain a higher incidence of the Clostridium histolyticum group of bacteria than that of healthy children [35, 36, 37].
On the other hand, low-grade endo-toxaemia has recently been observed in autism. Compared with healthy subjects, serum levels of bacterial endotoxin were significantly higher in autistic patients and inversely and independently correlated with the severity of autism symptoms, noting the need for further studies to establish whether increased endotoxin may contribute to the pathophysiology of inflammation and behavioural and social impairments in autism [38]. Presence in blood of endotoxins produced by gastrointestinal pathogens is indicative of impaired gastrointestinal permeability and present in chronic infectious and inflammatory conditions. A good example is the association of HIV-infection with increased gut permeability and microbial translocation, evidenced by increased circulating lipopolysaccharide (LPS) levels, mirroring the above-mentioned findings in autism [39].
3. Neurotransmitter abnormalities in Autism Spectrum Disorders
Abnormalities in neurotransmitter systems have frequently been recorded in autism. Clinical observations include both elevated and lowered levels of various neurotransmitters compared to controls, including alterations in monoamine metabolism [40, 41, 42], neurotransmitter peptides [43, 44], with considerably raised levels of beta-endorphin (for vasopressin/oxytocin see Hormones) and altered activities of cholinergic receptors, with binding of muscarinic M(1) receptor being up to 30% and that of nicotinic receptors being 65%-73% lower in the autistic group compared to controls [45]. Post-mortem brain examination noted abnormalities of the glutamate neurotransmitter system in autism, with specific abnormalities in the AMPA-type glutamate receptors and glutamate transporters in the cerebellum [46].
Dysregulations of serotonergic systems in particular have been documented, such as abnormalities in brain serotonin synthesis, with significant reductions in synthesis capacity compared to controls [47, 48], while at the same time plasma levels of serotonin and free tryptophan appear to be on average 30-50% percent higher in individuals with autism [49]. Autoantibodies to serotonin receptors [50] and reduced receptor binding have also been recorded [51]. Of note is that one study found correlation of elevated plasma serotonin levels and the major histocompatibility complex (MHC) types associated with autism [52].
Relative to expression and function of nicotinic receptors in autism, significantly lowered binding of some agonists to nicotinic receptors has been observed. For example, binding of the nicotinic acetylcholine receptor agonist, epibatidine in cortical areas to was up to 73% lower in autism group compared to controls. As with serotonin receptors, some of the nicotinic receptors have been noted to be significantly permeable to calcium and so able to regulate several neuronal processes. Preliminary reports of therapeutic action in autism of galantamine, an acetylcholinesterase, usually used for the treatment of cognitive decline in mild to moderate Alzheimer's disease and memory impairments[53, 54], are of interest considering that the neuroprotective actions of galantamine are thought to be linked to its modulation of nicotinic receptors [55].
A post-mortem study revealed significantly reduced levels of Glutamic Acid Decarboxylase (GAD65 and 67 kDa) proteins in several areas of the brains of individuals with autism [56]. This was confirmed by more recent results that showed GAD67 messenger RNA (mRNA) level wasreduced by 40% in the autistic group compared to controls [57]. (mRNA is made up of four molecular building blocks: adenosine, cytidine, guanosine and uridine, which can be modified by the attachment of a methyl tag. These tags or RNA modifications provide a sophisticated extra layer of epigenetic regulation. Hence the critical importance of methyl donors such as S-adenosyl Methionine, which in turn is dependent on the genes MTHFR, MTRR, MTR and MS to be functioning optimally, and on optimum levels of methyl B12 and methyl Folate).Another study found that serum levels of glutamate in the patients with autism were significantly higher than those of normal controls [58].
4. Hormonal abnormalities in autism spectrum Disorders
Abnormalities in hormonal metabolism are frequently observed in individuals with autism, with several studies observing abnormal levels of many hormones and their receptors compared to healthy controls, as well as abnormal hormonal secretion rhythms [59, 60, 61, 62]. For example, the analysis of the Hypothalamic-Pituitary-Adrenocortical (HPA) system responses observed more variable circadian rhythm as well as significant elevations in cortisol following exposure to a novel stimulus in children with autism compared to controls. This exaggerated cortisol response is indicative of dysfunction of the HPA system in autism [63]. Over-reaction of the endocrine system to insulin stress in autism has been recorded in another study, whereas the experimental stress of insulin-induced hypoglycaemia showed slower recovery of blood glucose, much faster cortisol response and elevation of growth hormone levels compared to controls [64, 65]. Low levels of insulin-like growth factor-I (IGF-I) in cerebrospinal fluid have also been observed [66].
Metabolic disorders of serotonin and dopamine systems have been suggested in autism, with around thirty percent of individuals with autism exhibiting high levels of serotonin, simultaneous with lowered levels of melatonin.Serotonin is converted to melatonin (important in the regulation of circadian rhythms that regulate the sleep/wake cycle).by several enzymes of the pinealocytes, cells in the pineal gland, including 5-HT N-acetyl transferase and 5-hydroxyindole-O-methyltransferase. Results of the studies looking at sleep disturbances in autism suggest that both dyssomnias and parasomnias are very prevalent in the disorders.People with autism frequently experience sleep disorders and exhibit atypical sleep architecture [67, 68, 69]. Further evidence of dysfunction of pineal endocrine system in autism was obtained by looking at alterations of the light and dark circadian rhythm of melatonin, where none of autistic patient showed a normal melatonin circadian rhythm, together with once again significantly lower levels of this hormone [70]. Leptin is a hormone linked to melatonin that plays an important role in amongst other things regulation of appetite and metabolism. Results from a recent study have demonstrated significant differences in leptin concentrations between children with autism and controls [71].
5. Auditory, visual, tactile and oral sensory processing disorders and motor difficulties in autism
Individuals with autism often present with auditory, visual, tactile and oral sensory processing disorders, as well as various forms of motor difficulties, including dyspraxia (occasionally linked to low muscle tone), dystonia (involuntary, sustained muscle contractions) and ataxia [72, 73, 74, 75, 76]. Visual disturbances in autism often include abnormalities of colour perception [77] and weak visual coherence. Retinal dysfunction in autism has been suggested, as well as deficits in visual processing in dorsal cortex [78, 79]. Abnormal pain perception is sometimes present in autism, as well as self-injurious behaviour(possibly. In part due to COMT gene mutation).
Dyspraxia is a disorder of coordination that can also be described as a difficulty with planning a sequence of coordinated movements, or in the case of ideo-motor dyspraxia, a difficulty with executing a known plan. Various areas of difficulty can include speech and language, fine motor control (e.g. handwriting or holding pencil in a correct way), poor spacial awareness and timing and balance of body movements and difficulty combining movements, poor physical play skills (throwing and catching a ball) and difficulty in manipulating small objects. Ataxia refers more specifically to a failure of muscle control in limbs, often resulting in a lack of balance and coordination and abnormal gait [81, 82, 83].
Movement is coordinated by the cerebellum and its connections to the motor cortex, which requires adequate myelination of neurons in white matter and optimum functioning of cells in grey matter. Both require DHA from deep-sea cold-water fish. The dry volume of brain cells in all healthy mammals contains around 30% Omega 3 (EPA+DHA).
6. Reduced cerebral blood flow and cerebral oedema in autism Spectrum Disorders
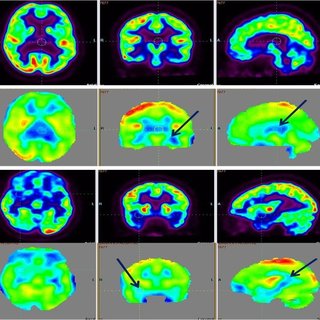
Results of several studies have shown abnormal platelet reactivity and altered blood flow in children with autism. Following these findings, it has been suggested that platelet and vascular endothelium activation could be one of the contributing factors to the development and clinical manifestations of the disorder [84]. Relative to these the following case reports are of particular interest, both describing cases of inflammation of brain blood vessels resulting in loss of language and emergence of symptoms of autism. In both cases administration of nicardipine lead to recovery of language and behaviour [85, 86].
PET and SPECT scans in autistic children show a decreased cerebral blood flow in some regions of the brain [87, 88, 89] and cerebral water content was found to be raised in brain grey matter in children with autism [90]. A model has been suggested in which the observed grey matter abnormality could be inflammatory. This finding of cerebraloedema at the same time offered an alternative explanation for enlarged brain size in autism, which up to then had been hypothesised to be due to lack ‘pruning’ of neurons during development.
7. Abnormality of the immune function and chronic inflammation in autism Spectrum Disorders
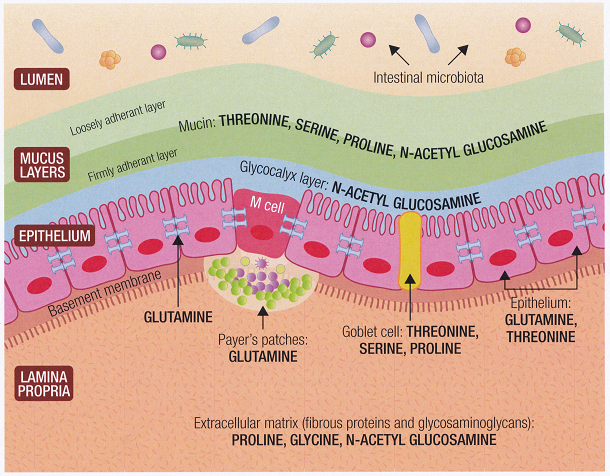
Results of numerous studies point to an abnormality of the immune function in autism, as well as active, ongoing inflammation in the GI tract, the brain and the cerebrospinal fluid (CSF). A recent study [91] investigated the presence of immune activation in post-mortem brain specimens and CFS from subjects with autism. The authors found active neuroinflammation in multiple areas of the brain, for example in the cerebral cortex and white matter, and in the cerebellum. A marked microglial and astroglial activation was also found, as well as the presence of an altered cytokine pattern, with macrophage chemoattractant protein (MCP)-1 and tumour growth factor-beta1 (TGF-beta1) being the most prevalent cytokines. There was also an accumulation of macrophages and monocytes, and a marked absence of lymphocytes and antibodies, pointing toward an innate neuroimmune activation with the absence of adaptive immune system/T cell activation in the brain. In addition, an enhanced proinflammatory cytokine profile was observed in the CSF, including once more a marked increase in MCP-1 [91]. These observations resemble findings in other neurological disorders in which elevations in cytokine levels is associated with the pathogenesis of neuroinflammation, neurotoxicity and neuronal injury and subsequent behavioural and cognitive impairments, for example HIV-associated dementia and multiple sclerosis [92, 93, 94, 95]. Animal experiments illustrate that, during early pre and postnatal development, inflammatory cytokine challenge can induce various psychological, behavioural and cognitive impairments [96, 97, 98]. At the same time the expression of many cytokines, including MCP-1, in neurons and glial cells seems to be upregulated by increased intracellular calcium triggered by membrane depolarisation [99, 100].
Another investigation into inflammatory markers in the brain tissue of patients with autisms revealed significantly increased levels of several proinflammatory cytokines (TNF-alpha, IL-6 and GM-CSF, IFN-gamma, IL-8). The Th1/Th2 ratio was also significantly increased in ASD patients, suggesting that localized brain inflammation and autoimmune disorder may be involved in the pathogenesis of ASD [101].
Various serological findings further confirm the presence of immune system dysregulation and active inflammation in autism - raised levels of proinflammatory cytokines have often been observed in blood of patients with autism, with significant increases of IFN-gamma, IL-6 and TNF-alpha. These results are followed by findings of decreased peripheral lymphocyte numbers, incomplete or partial T cell activation following stimulation, decreased NK cells activity, dysregulated apoptosis mechanisms, imbalances of serum immunoglobulin levels, increased numbers of monocytes and abnormal T helper cell (Th1/Th2) ratio, with a Th2 predominance, and without the compensatory increase in the regulatory cytokine IL-10 [101a, 102a]. It is of interest to note that, following increased levels of TGF-beta1 in brain specimen as observed by Vargas et al, this cytokine was found to be significantly lower in the blood of adult patients with autism compared to controls [102].
Another relevant observation is the elevation of cerebrospinal fluid levels of TNF-alpha compared toits serum levels in subjects with autism. The observed ratio of 53.7:1 is significantly higher than the elevations reported for other pathological states for which cerebrospinal fluid and serum tumour necrosis factor-alpha levels have been simultaneously measured [103].
It has been suggested that prenatal viral infection might dysregulate foetal immune system, resulting in viral tolerance in autism [104]. Studies showing altered T-cell subsets raised suspicions about possible autoimmune aspects of autism and several studies pointed to association of the risk of autism to immune genes located in the human leukocyte antigen (HLA) [105, 106]. The immune system uses the HLAs to differentiate self-cells and non-self-cells and some of them are linked to higher risks of autoimmune disorders.
Animal studies show that behavioural changes follow onset of autoimmune disease and can be reversed through immune-suppressive treatments [107] and although various autoantibodies to brain antigens have been observed in autism, the results of those studies are often conflicting. In addition to the inconsistent findings the question has been raised as to whether those autoantibodies would be pathogenic contributors or mere consequences of the disorder. Of note in this context is the finding pointing to a possible association of virus serology and brain autoantibodies in autism [108]. One study found that children with autism had a significantly higher percent seropositivity of anti-nuclear antibodies. Anti-nuclear antibody seropositivity was significantly higher in autistic children with a family history of autoimmune disorders than those without such history, and also had significant positive associations with disease severity, mental retardation and electroencephalogram abnormalities [109]. Autistic children were found to have significantly higher serum anti-myelin-associated glycoprotein antibodies than healthy children. Family history of autoimmunity in autistic children was significantly higher than controls. Anti-myelin-associated glycoprotein serum levels were significantly higher in autistic children with than those without such history [110].
A study looking at several markers of concomitant autoimmunity and immune tolerance found highly elevated circulating IgA and IgG autoantibodies to casein and gluten dietary proteins in autism sample compared to controls. Circulating anti-measles, anti-mumps and anti-rubella IgG were positive in only 50%, 73.3% and 53.3% of autistic children previously immunised by MMR, as compared to 100% positivity in the control group. Anti-cytomegalovirus CMV IgG was also investigated and was positive in 43.3% of the autistic children as compared to 7% in the control group [111].
A recent study found polyomavirus infection in post-mortem autistic brains BKV, JCV, and SV40 viruses were significantly more frequent among autistic patients compared to controls [112]. Also, worth noting is the finding that a large subset of subjects with autism shows evidence of bacterial and/or viral infections not present in age-matched controls [113].
8. Oxidative stress in Autism Spectrum Disorders
Oxidative stress is defined as an imbalance between pro-oxidants and anti-oxidants, resulting in damage to cell by reactive oxygen species (ROS). During times of environmental stress ROS levels can increase dramatically which can result in significant damage to cell structures, especially in absence of anti-oxidant defences, such as the enzymes superoxide dismutase, catalase, glutathione peroxidase and glutathione reductase or antioxidant vitamins A, C and E and polyphenol antioxidants. There is mounting evidence that abnormalities of ROS and nitric oxide (NO) may underlie a wide range of neuropsychiatric disorders. Abnormal methionine metabolism, high levels of homocysteine and oxidative stress are also generally associated with neuropsychiatric disorders. NO signalling has been implicated in a number of physiological functions such as noradrenaline and dopamine releases. NOis thought to have neuroprotective effects at low to moderate concentrations, but excessive NO production can cause oxidative stress to neurons thus impairing their function.
Studies comparing the level of homocysteine and other biomarkers in children with autism to controls showed that in children with autism there were higher levels of homocysteine, which was negatively correlated with glutathione peroxidase activity, low human paraoxonase 1 arylesterase activity, suboptimal levels of vitamin B 12 [114, 115] and increased levels of NO [116, 117].
Lipid peroxidation was found to be elevated in autism indicating increased oxidative stress. Moderate to dramatic increases in isoprostane levels [118, 119], decreased levels of phosphatidylethanolamine and increased levels phosphatidylserine [120] were observed in children with autism as compared to controls. Levels of major antioxidant proteins transferrin (iron- binding protein) and ceruloplasmin (copper-binding protein) were found to be significantly reduced in sera of autistic children. A strong correlation was observed between reduced levels of these proteins and loss of previously acquired language skills [121].
Another study measured levels of metabolites in methionine pathways in autistic children and found that plasma methionine and the ratio of S-adenosylmethionine (SAM) to S-adenosyl-homocysteine (SAH), an indicator of methylation capacity, were significantly decreased in the autistic children relative to controls. In addition, plasma levels of cysteine, glutathione, and the ratio of reduced to oxidized glutathione, indicative of antioxidant capacity and redox homeostasis, were significantly decreased in autistic group. The same study evaluated common polymorphic variants known to modulate these metabolic pathways in 360 autistic children and 205 controls. Differences in allele frequency and/or significant gene-gene interactions were found for relevant genes encoding the reduced folate carrier (RFC 80G), transcobalamin II (TCN2 776G), catechol- O-methyltransferase (COMT 472G), methylenetetrahydrofolate reductase (MTHFR 677C and 1298A), and glutathione-S- transferase (GSTM1) [122].
Oxidative damage in autism is also associated with altered expression of brain neurotrophins critical for normal brain growth and differentiation. An increase in 3-nitrotyrosine (3-NT), a marker of oxidative stress damage to proteins in autistic cerebella has been reported. Altered levels of brain NT-3 are likely to contribute to autistic pathology not only by affecting brain axonal targeting and synapse formation but also by further exacerbating oxidative stress and possibly contributing to Purkinje cell abnormalities [123].
A study looking into cellular and mitochondrial glutathione redox imbalance in lymphoblastic cells derived from children with autism found that, compared to controls, autism LCLs exhibit a reduced glutathione reserve capacity in both cytosol and mitochondria that may compromise antioxidant defence and detoxification capacity under prooxidant conditions [124].
9. Mitochondrial dysfunction in Autism Spectrum Disorders
Mitochondrial dysfunction with defects in oxidative phosphorylation has been suspected in autism and several recent findings that show abnormalities in mitochondrial enzyme activities that support the hypothesis. Post-mortem examination of autistic brains revealed significantly elevated calcium levels in autistic brains compared to controls, followed by elevations of mitochondrial aspartate/glutamate carrier rates and mitochondrial metabolism and oxidation rates [125].
When compared to controls autistic patients show significantly lower carnitine levels, followed by elevated levels of lactate, aspartate aminotransferase, creatine kinase and significantly elevated levels of alanine and ammonia [126, 127, 128]. A pilot study investigating brain high energy phosphate and membrane phospholipid metabolism in individuals with autism found decreased levels of phosphocreatine and esterified ends (alpha ATP + alpha ADP + dinucleotides + diphosphosugars) compared to controls. When the metabolite levels were compared with neuropsychologic and language test scores, a common pattern of correlations was observed across measures in the autistic group, wherein as test performance declined, levels of high energy phosphate compounds and of membrane building blocks decreased, and levels of membrane breakdown products increased. The authors concluded that the results of the study provided tentative evidence of alterations in brain energy and phospholipid metabolism in autism that correlate with the level of neuropsychologic and language deficits [129]. This was further confirmed by another study finding the impairment of energy metabolism in autistic patients which could be correlated to oxidative stress [130].
10. What does it all mean and what can be done?
There is general consensus that Autism Spectrum Disorder is caused by an interaction between de-novo (new) genetic mutations and the environment. In all likeliness, the genetic mutations are due to toxins in food, in the air we breathe and in the environment. These are mostly petrochemical derivatives used as food colourings, additives, preservatives, pesticides, cleaning compounds, smoke from chimney stacks, plastics, and a myriad of other products. These find their way into the placenta of expecting mothers and passed on to cord blood of babies. A study of 10 random cord blood samples found 287 toxic elements, an average of 200 per sample. 121 of these were from products that had been banned for more than 30 years. Many of these are individually known to damage DNA and cause cancer, and their combination is thought to be even more damaging (131, 132]. The decline of the nutrient contents of the food that we eat has been well documented [133, 134]. The food that we eat is grown in nutrient depleted soils, and chemical fertilisers are used to speed up growth and yield, with no regard for nutrient contents.
In 1960, a new genetically engineered and genetically modified wheat variety, Triticum Aestivum, was released on the world market. It is a high yield, high protein and pest resistant variety designed to put an end to world famine. Its high pest resistance comes from its high levels of wheat germ agglutinin (WGA) a lectin which binds to two sugars in the cell membranes of insects, destroying the cell membranes and killing the insect.
Unfortunately, all animals including humans use these sugars (N-Acetyl-glucosamine and N-acetyl-Neuraminic acid) as basic building blocks of our cell. All epithelial cells contain high levels of N-Acetyl-glucosamine, ear, nose, throat, lungs, gastrointestinal track, joints, arteries (particularly around the heart) and these tissues are all damaged by WGA, with evidence of increased inflammation and tissue damage[135, 136, 137, 138].
Our genes have adapted to make proteins that repair tissues damaged by WGA after thousands of years of exposure to wheat. However, people in countries who have only recently been exposed to wheat (less than 80 years for Asian populations) may have less adaptation. Also, we now consume far more WGA in our “western style diet”; hence, if the genes responsible for repairing various types of epithelial cells mutate from exposure to toxins, we may be less able to carry out the repair and be more prone to disease [137].
All of the Biomedical abnormalities that we have listed above in ASD are contributed by these three factors:
-
De novo mutations from toxins.
-
Environmental toxins (including WGA) causing inflammation.
-
Reduced nutrient levels in our food chain.
Consequently, there has been an exponential increase in non-communicable diseases and our health, that of our children and that of future generations are at the mercy of toxins and the western style diet. Since mutations that cause genetic predispositions are passed down to future generations, no wonder, in 2011 the Director General of the WHO calledthis a slow motion catastrophe, an impending disaster, warning that shortly not even the wealthiest countries in the world will be able to cope and afford their health bill [138]
Optimal treatment for ASD is to first address the causes of these Biomedical abnormalities and to continuously support the recovery and health of the brain and other tissues Through diet and nutrient supplementation. Second, Early Intensive Behaviour Intervention, to help optimise behaviours and finally to use Neurotherapy to retrain the brain, and improve concentration and compliance and help normalise behaviours.